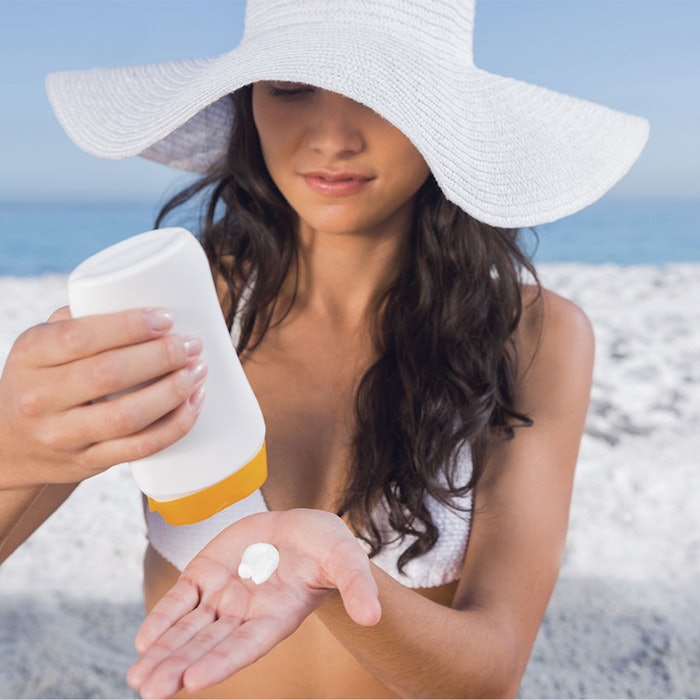
The photochemistry of avobenzone has extensively been studied; in particular, its compatibility with other organic molecules, in different matrices, in biological systems and its ability to provide effective photoprotection.15 These considerations are the main focus of this second article in a two-part series on avobenzone; part one appeared in the Cosmetics & Toiletries October 2017 issue.
Log in to view the full article
The photochemistry of avobenzone has extensively been studied; in particular, its compatibility with other organic molecules, in different matrices, in biological systems and its ability to provide effective photoprotection.15 These considerations are the main focus of this second article in a two-part series on avobenzone; part one appeared in the Cosmetics & Toiletries October 2017 issue.
Avobenzone with UV Filters
Table 1 shows how different molecules can influence the photodegradation of avobenzone; following is a discussion.28
Octinoxate (syn. octyl methoxy cinnamate or ethylhexylmethoxycinnamate): Octinoxate’s structure and absorption spectrum in cyclohexane are shown in Figure 9a. This molecule is an excellent UVB filter. Octinoxate can quench the triplet state of the keto form of avobenzone with a rate constant of 7.3 × 109 M-1s-1,29, 30 and thereby should stabilize avobenzone.
However, the triplet form of octinoxate formed via the quenching of photo-excited avobenzone can undergo photoisomerization, minimizing its photoprotection effect.31 Octinoxate also does not exhibit reactivity with singlet oxygen. In the case of octinoxate alone, the issue is trans to cis photoisomerization and its innate photoreactivity ([2+2] photocycloaddition between two cinnamate molecules).32, 33
In addition, bimolecular photoreactions with the enolic form of avobenzone (Mayo reaction), leading to cyclobutylketone adducts and subsequent photodegradation, have been observed.34, 35 Due to the bimolecular nature of these degradation reactions, the concentrations of molecules during photolysis is important.36
Octocrylene: The structure and UV/visible spectrum of octocrylene is shown in Figure 9b. Octocrylene is photostable and possibly the best molecule for photostabilizing avobenzone.28, 36 Experiments performed with the methylated form of avobenzone, where only the keto form is present, indicate a rate constant for quenching the triplet state at 3.9 × 109 16 M-1s-1.29 The triplet form of octocrylene is also stable against photodecomposition.
Further, the reactivity of octocrylene with singlet oxygen is moderate (kq of ~106 M-1s-1).29 Thus, the efficacy of octocrylene in protecting against the photodecomposition of avobenzone arises from both quenching the triplet form of its keto isomer and octocrylene’s own photostability. Ethylhexylmethoxcrylene, which is related to octocrylene, has been reported to quench the singlet excited state of the enolic form. Note this trait was determined by a change in fluorescence lifetime; data was not analyzed to obtain a quenching constant.12
Dioctyl butamido triazone (DBT; also iscotrizinol): Figure 10a shows the molecular structure of DBT. This material also improves the photostability of avobenzone by quenching the singlet oxygen produced from the triplet state of the keto form of avobenzone, with a rate constant of 1.8 × 108 M-1s-1—without being degraded.29
Bis-ethylhexylphenol methoxyphenol triazine (also bemotrizinol or benzotrizinol): Figure 10b shows the structure of this molecule, which also is believed to photostabilize avobenzone.29, 37 bis-Ethylhexyloxyphenol methoxyphenyl triazine is proposed to act by quenching avobenzone’s triplet state and remain stable against photodecomposition; it does not quench singlet oxygen.37
Antioxidants: The photochemistry of avobenzone in the presence of different antioxidants has been reported as well. For example, with vitamins E and C and ubiquinone, in solutions of avobenzone in DMSO, some stabilization was observed but in this case, it did not scale with the concentration of antioxidant used.38
In another study, α-tocopherol (of the vitamin E group) was incorporated with avobenzone into lipid-based nanoparticles using melt emulsification. Absorption spectra showed an increase in avobenzone intensity at 360 nm with irradiation but it is unclear how the intensity could increase—i.e., if avobenzone is stabilized, its absorption spectra should remain constant. These researchers claimed, however, that the stability provided to avobenzone was due to antioxidants scavenging reactive oxygen species; although no direct experimental proof of this was provided.39
On the other hand, with the plant antioxidant rutin and avobenzone incorporated in o/w emulsions, rutin apparently did not alter the photostability of avobenzone.40 In another study with the naturally occurring antioxidant flavonoid quercetin, o/w emulsions with avobenzone were prepared.41 Under the photolysis conditions, avobenzone alone degraded by 40.3%, while in the presence of 0.5% w/w quercetin, its photodegradation decreased to 27.7%. This improvement in avobenzone photostability was, again, attributed to antioxidants quenching reactive oxygen and avobenzone’s triplet state.41
Doped TiO2: Finally, manganese-doped TiO2 (Mn-TiO2) also has been suggested to stabilize avobenzone.42, 43 With doped Mn, an increase in UVA absorption is observed, as is a reduction in reactive oxygen free radical generation—by 97% due to electron-hole de-excitation via the Mn and free radical scavenging. Avobenzone was photodegraded down to 63% in the absence of titania but improved to 79% in the presence of Mn-TiO2 (10 mW/cm2 of simulated solar radiation for 2 hr). Similar results were observed with mixtures of avobenzone and octinoxate.43
Thus, taken together, the stabilization of avobenzone is possible with additives that:
- Do not react with it under photochemical conditions (octinoxate does; octocrylene, bemotrizinol and DBT do not).
- Are efficient quenchers of avobenzone’s triplet state (e.g., octocrylene).
- Are photostable on their own (e.g., octocrylene, triazines and triazone).
- Quench singlet oxygen (e.g., triazones).
- Are not degraded by singlet oxygen
(e.g., octocrylene, triazones). - Have some overlap with both UV-A and -B spectra, so that photons are absorbed, minimizing decomposition (e.g., triazines, triazones).
- Provide singlet-singlet quenching by molecules to retard avobenzone photodestruction (e.g., ethylhexylmethoxcrylene, diethylhexyl-2, 6-napthalate and diethylhexylsyringylidene).
- Include antioxidants and doped TiO2, which trap free radicals.
Avobenzone in Different Matrices
The photochemistries of avobenzone entrapped in polymeric nanoparticles, lipid particles and cyclodextrins have been reported.44 Polyamide-based nanocapsules and nanoemulsions were used to entrap avobenzone. From both of these substrates, avobenzone was released—37% from nanocapsules and 95% from nanoemulsions.45 Photodegradation within 10 min after encapsulation (< 3% of avobenzone released) also showed that both the released and encapsulated avobenzone were photodegraded. The amount of degradation depended on the photon flux. For example, with 0.5 J/cm2 (20 MED), ~20% of the avobenzone in nanocapsules was degraded, compared with 40% non-encapsulated or in the nanoemulsion.
Lipid microparticles formed with a solid lipid core surrounded by a layer of surfactant molecules has also been used to entrap avobenzone via a hot emulsion technique.46 Here, avobenzone escaped from these particles into propylene glycol. The kinetics of release varied with the lipid composition. The best results were obtained with glyceryl behenate and poloxamer, with ~80% release in 2 hr. After just 1 hr of simulated solar radiation illumination, free avobenzone/octinoxate/octocrylene showed a degradation of 28.6%, versus lipid microparticle avobenzone/free octinoxate/octocrylene at 26%, and lipid microparticles of avobenzone with octocrylene at 21.5%.46
The photodegradation of avobenzone decreased in emulsions prepared with the naturally occurring antioxidant quercetin.
The photochemistry of avobenzone nanoparticles, avobenzone trapped in polymethylmethacrylate (PMMA)—both prepared by rapid injection of a tetrahydrofuran (THF, also known as oxolane) solution into water, and avobenzone dissolved in THF were compared.47 The photodegradation, as measured by UV-visible spectroscopy for 60 min, indicated avobenzone degradation was similar in THF and PMMA, while the avobenzone nanoparticles exhibited more degradation (as estimated from the areas under the absorption bands).47 In this study, it was unclear why the authors concluded PMMA stabilized the avobenzone.
Silica glass microcapsules prepared by the sol-gel method with a size distribution of 0.3–3 microns also has been used to encapsulate avobenzone. This study did not report on the photostability of avobenzone; the focus of the encapsulation was to decrease leaching and avoid contact with the skin.48
There are also several reports on the encapsulation of avobenzone in cyclodextrins.49, 50 Complexation with hydroxypropyl-β-cyclodextrin (HPCD) was reported to photostabilize avobenzone, and the free radicals released upon photolysis of the avobenzone-HPCD complex was lower than free avobenzone, presumably due to the cyclodextrin trapping the radicals or radical recombination in the encapsulated state.50
In another study, HPCD (20%) was found to uptake ~0.12 mg/mL of avobenzone from aqueous solutions. Upon encapsulation, there was a slight blue shift in the absorption spectra, possibly due to the formation of an inclusion complex. With 30% HPCD, the avobenzone was most stable, reaching a recovery rate of 79.3% after 50 J/cm2 of > 290 nm radiation for 80 min; without HPCD, the degradation was 16.6%. Lower radiation dosage (up to 10 J/cm2) did not alter the recovery with and without HPCD.49
Finally, zeolites are microporous aluminosilicates with cages and channels of molecular dimensions. Several organic filters have been incorporated into zeolites, including p-aminobenzoic acid and oxybenzone. Incorporation into zeolites reduced biological damage as well as reduces contact with the skin although there are no reports of avobenzone encapsulation by zeolites.51–53
Based on these studies of encapsulating avobenzone in different matrices, shown schematically in Figure 11, it can be surmised that:
- The main benefit of encapsulation is that the avobenzone is isolated from its surroundings, including the skin.
- Leakage from the encapsulating matrix is relevant.
- There can be some protection from photodecomposition, though why that is the case is not clear. The most likely explanation could be the polarity of the encapsulating medium, which promotes the reformation of the enol from the photo-excited state.
Avobenzone in Biological Systems
Irradiated mixtures of DNA and avobenzone result in strand breaks, i.e., plasmid nicking of the DNA, caused by the reaction of the carbon-centered radicals (or peroxyl radicals) from the photodecomposition of avobenzone with DNA.25 Such DNA breaks can be avoided in the presence of piperidine nitroxide or indolinic nitroxide radicals since these react with the carbon-centered radicals.25
Carbonyl formation in bovine serum albumin, indicative of oxidative damage, also has been shown to increase with both time of irradiation and concentration of avobenzone, and are attributed to the formation of carbon-centered radicals formed upon photodecomposition of avobenzone.54
However, avobenzone mixed with Chinese hamster ovarian cells and subjected to irradiation did not increase the photoclastogenic activity.55 In addition, a protective effect on human lymphoid cells was noted in the presence of avobenzone upon UVA/UVB irradiation.14 Thus, it appears the photobiology of avobenzone is controlled by the formation of the radicals from the photoexcited keto state.
The photobiology of avobenzone appears to be controlled by the formation of the radicals from the photoexcited keto state
Photo-allergy and Other Biological Interactions
Several reports have indicated photo-allergies in relation to organic filters that include avobenzone.56–65 Thus, the toxicity of avobenzone has recently been reviewed.66 A substance applied to the skin is considered phototoxic if a non-immunological toxic response, e.g., dermatitis such as erythema, edema, vesiculation and pigmentation, is observed after exposure to light. Such phototoxic responses do not require an induction period.67 Photoallergic contact dermatitis arises when light induces the formation of a hapten or antigen, which can trigger the allergic response;67 however, it is difficult to estimate the prevalence of these effects.
In one European study of 1,031 patients exposed to 19 organic UV absorbers and five nonsteroidal anti-inflammatory drugs, 200 patients (19.4%) tested positive to photoallergic contact dermatitis as measured by the photopatch test. Eighteen patients reacted negatively to avobenzone and 41 to octocrylene, which is often used to photostabilize avobenzone.63
Avobenzone photoproducts, in particular the arylglyoxals, were observed to be strong sensitizers in a mouse local lymph node assay.68 Avobenzone also exhibited phototoxicity potential as detected by the 3T3 Neutral Red Uptake Phototoxicity test.67 However, the phototoxicity was not observed when tested on a human 3-D skin model, possibly because it did not penetrate the skin.67
In a year-long test on hairless mice, avobenzone or its photoproducts were found to protect against UV-induced skin tumor formation.69 In another study, avobenzone alone or in combination with octinoxate was applied to Skh-1 albino hairless mice and irradiated with full spectrum or UVA for 8 hr daily, five days/week, for 16–32 weeks. At 2% avobenzone (oil/water emulsion), reduced photodamage to connective tissue was observed. At the lowest 0.75% level of avobenzone, no photoprotective effect was noted.
Mixtures of organic molecules (UV filters) can also enhance sensitization.6, 70 For example, amongst cosmetic products such as sunscreens, hair styling products, lip cosmetics and perfumes, sunscreens are responsible for majority of cases of photoallergic contact dermatitis, stemming from high concentrations and mixtures of UV filters. Avobenzone is the most widely used UV filter in sunscreens; the second most used in cosmetics (after octinoxate). Thus, not surprisingly, it yields the most positive photopatch test reactions.
Octocrylene, which has been associated with photoallergy to ketoprofen, also results in a large number of photoallergy reactions.70 In most formulations involving avobenzone, octocrylene is added to improve photostability due to the reasons discussed above. An issue with octocrylene, however, is that it is both a photocontact and contact allergen,70 with the latter being prevalent more in children.71
In vitro studies of avobenzone showed no increase in cell proliferation of MCF-7 breast cancer cells. Also, uterotrophic assays conducted by feeding avobenzone to immature Long-Evans rats exhibited no effect. Therefore, avobenzone is not considered an agent for endocrine activity, though other commonly used filters such as octinoxate can exhibit some activity.72
All in all, the toxicity of avobenzone appears to arise from the large number of photo decomposed products and their accumulation on the skin. Cellular culture studies suggest avobenzone itself is non-toxic.
Relevance to Sun Protection
A review of cosmetic products in the United Kingdom showed UV filters are present in a wide variety of cosmetics; avobenzone was in some 48.7% of 4,447 products.6 Several studies have reviewed the use of avobenzone as an organic filter for sunscreens.3, 73, 74 In most practical applications, triplet quenchers such as octocrylene, cinnamates and 4-methylbenzylidene camphor are included with avobenzone, as noted above, to minimize photodegradation channels from the triplet keto state. Compounds such as octinoxate and 2-ethylhexyl-4-dimethyl-aminobenzoate (padimate O) must be avoided in formulations with avobenzone since these molecules react with it in the presence of sunlight.75
The toxicity associated with avobenzone appears to arise from its photodecomposition products, not avobenzone itself.
In one study, the photodegradation of avobenzone present in a variety of commercial sunscreens was examined using a solar simulator and its extent varied depending on the additives use. Overall, the SPF decreased in cases where the avobenzone was not stabilized.18 This study pointed out that frequent application of sunscreens that decompose can address inadequate photoprotection but that the photodegraded products still build up on the skin surface.18
Finally, the U.S. Food and Drug Administration guidelines do not allow mixtures of avobenzone with TiO2 or ZnO,75 possibly because avobenzone is unstable in their presence (both anatase and rutile TiO2). This metal oxide-mediated degradation can be slowed if the TiO2 or ZnO are coated with silanes, methicones, stearic acid, long-chain fluoro compounds or lignins.76
Thus, for the practical application of avobenzone as a sunscreen:
- It must be photostabilized.
- Its decomposed photoproducts can be toxic and photoallergenic, and they accumulate on skin.
- The molecules added to stabilize avobenzone can also be photoreactive and phototoxic.
Conclusion: Parts I and II
Avobenzone exists primarily as the enol isomer and its photochemistry is controlled by three rotamers formed via rotations around single and double bonds connecting the two benzene rings in the dibenzoyl derivative. The excited state dynamics are complicated, with two of the rotamers formed via single bond rotation, returning to the enol on picosecond time scales.
However, the species formed via isomerization around the double bond is long-lived (milliseconds) and can transform into the keto form of avobenzone. This conversion to the keto form is controlled by the polarity of the solvent and favored in nonpolar media.
The keto form has its own photochemistry, driven primarily by a long-lived triplet state, which can undergo photodegradation and form singlet oxygen. This is the primary channel for photodegradation of avobenzone. In the initial step of the photodegradation of the keto form, carbon-centered free radicals are formed. These reactive radicals can combine to form numerous products, most of them absorbing in the UVC range.
For practical applications with avobenzone, it is essential that the molecule be photo-protected, which is typically accomplished by the addition of other organic molecules primarily to quench the triplet state of the keto form. Encapsulation strategies have been attempted to provide photostability with little success due to either the leakage of avobenzone or photochemistry within the encapsulant.
Avobenzone has excellent absorption properties in the UVA region and provides significant health benefits as a sunscreen agent. Based on in vitro studies, it also appears to be nontoxic. The disadvantages associated with avobenzone include the accumulation/toxicity of its photoproducts, and/or the toxicity of molecules added to stabilize avobenzone.
Finally, long-term stability is a potential possibility if entrapment does not allow for isomerization around the double bond. This will require strict spatial control but also lengthen the time of photo-protection afforded by avobenzone.
Acknowledgments: The authors wish to acknowledge helpful discussions with Steve Jones, CEO of ZeoVation; David Bergeron, VP, Rev 1; and Jay Dahlman, Ph.D., of Ohio State University.
References
Continued from Part I
- C Stiefel and W Schwack, Photoprotection in changing times–UV filter efficacy and safety, sensitization processes and regulatory aspects, Intl J Cosm Sci 37 (1) 2–30 (2015) doi: 10.1111/ics.12165
- W Uter, M Gonçalo, K Yazar, E-M Kratz, G Mildau and C Lidén, Coupled exposure to ingredients of cosmetic products: III. Ultraviolet filters, Contact Dermatitis 71(3) 162–169 (2014) doi: 10.1111/cod.12245
- I Andrae et al, A UVA filter (4-tert-butyl-4′-methoxydibenzoylmethane): Photoprotection reflects photophysical properties, J Photochem Photobiol 37(1) 147–150 (B 1997) doi: 10.1016/S1011-1344(96)07330-7
- PK Verma, F Koch, A Steinbacher, P Nuernberger and T Brixner, Ultrafast UV-induced photoisomerization of intramolecularly H-bonded symmetric β-diketones, J Am Chem Soc 136 (42) 14981–14989 (2014) doi: 10.1021/ja508059p
- SP Huong, E Rocher, J-D Fourneron, L Charles, V Monnier, H Bun and V Andrieu, Photoreactivity of the sunscreen Butylmethoxydibenzoylmethane (DBM) under various experimental conditions, J Photochem Photobiol Chem 196(1) 106–112 (2008) doi: 10.1016/j.jphotochem.2007.11.023
- U Osterwalder and B Herzog, The long way toward the ideal sunscreen—Where we stand and what still needs to be done, Photochem Photobiol Sci Off J Eur Photochem Assoc Eur Soc Photobiol 9(4) 470–481 (2010) doi: 10.1039/b9pp00178f
- V Lhiaubet-Vallet et al, Filter-Filter interactions. Photostabilization, triplet quenching and reactivity with singlet oxygen, Photochem Photobiol Sci Off J Eur Photochem Assoc Eur Soc Photobiol 9(4) 552–558 (2010) doi: 10.1039/b9pp00158a
- A Kikuchi and M Yagi, Direct observation of the intermolecular triplet-triplet energy transfer from UV-A absorber 4-tert-butyl-4'-methoxydibenzoylmethane to UV-B absorber octyl methoxycinnamate, Chem Phys Lett 513(1–3) 63–66 (2011) doi: 10.1016/j.cplett.2011.07.067
- A Kikuchi, Y Nakabai, N Oguchi-Fujiyama, K Miyazawa and M Yagi, Energy-donor phosphorescence quenching study of triplet–triplet energy transfer between UV absorbers, J Lumin 166 203–208 (2015) doi: 10.1016/j.jlumin.2015.05.033
- N Serpone, A Salinaro, AV Emeline, S Horikoshi, H Hidaka and J Zhao, An in vitro systematic spectroscopic examination of the photostabilities of a random set of commercial sunscreen lotions and their chemical UVB/UVA active agents, Photochem Photobiol Sci Off J Eur Photochem Assoc Eur Soc Photobiol 1(12) 970–981 (2002)
- A Schrader, Photochemical studies on trans-3-methylbutyl 4-methoxycinnamate—Abstract, J Cosmet Sci 45(1) 43–52 (1994)
- RM Sayre, JC Dowdy, A Gerwig, WJ Shields and RV Lloyd, Unexpected photolysis of the sunscreen octinoxate in the presence of the sunscreen avobenzone, Photochem Photobiol 81(2) 452–456 (2005) doi: 10.1562/2004-02-12-RA-083
- W Johncock, Formulation of sunscreens. Favorable and unfavorable interactions, Cosmet Technol 3(5) 25–31 (2000)
- B Herzog, M Wehrle and K Quass, Photostability of UV absorber systems in sunscreens, Photochem Photobiol 85(4) 869–878 (20089) doi: 10.1111/j.1751-1097.2009.00544.x
- E Chatelain and B Gabard, Photostabilization of butyl methoxydibenzoylmethane (avobenzone) and ethylhexyl methoxycinnamate by bis-ethylhexyloxyphenol methoxyphenyl triazine, a new UV broadband filter, Photochem Photobiol 74(3) 401–406 (2001)
- S Afonso et al, Photodegradation of avobenzone: Stabilization effect of antioxidants, J Photochem Photobiol B 140 36–40 (2014) doi: 10.1016/j.jphotobiol.2014.07.004
- G Niculae, I Lacatusu, A Bors and R Stan, Photostability enhancement by encapsulation of a-tocopherol into lipid-based nanoparticles loaded with a UV filter, Comptes Rendus Chim 17(10) 1028–1033 (2014) doi: 10.1016/j.crci.2013.12.007
- CA de Oliveira et al, Functional photostability and cutaneous compatibility of bioactive UVA sun care products, J Photochem Photobiol B 148 154–159 (2015) doi: 10.1016/j.jphotobiol.2015.04.007
- A Scalia and M Mezzena, Photostabilization effect of quercetin on the UV filter combination, butyl methoxydibenzoylmethane-octyl methoxycinnamate, Photochem Photobiol 86(2) 273–278 (2010) doi: 10.1111/j.1751-1097.2009.00655.x
- G Wakefield, S Lipscomb, E Holland and J Knowland, The Effects of manganese doping on UVA absorption and free radical generation of micronized titanium dioxide and its consequences for the photostability of UVA absorbing organic sunscreen components, Photochem Photobiol Sci Off J Eur Photochem Assoc Eur Soc Photobiol 3(7) 648–652 (2004) doi: 10.1039/b403697b
- G Wakefield and J Stott, Photostabilization of organic UV-absorbing and antioxidant cosmetic components in formulations containing micronized manganese-doped titanium oxide, Int J Cosmet Sci 29(2) 139–139 (2007) doi: 10.1111/j.1467-2494.2007.00369_1.x
- C Cerqueira Coutinho, EP dos Santos and CRE Mansur, Nanosystems in photoprotection, J Nanosci Nanotechnol 15(12) 9679–9688 (2015) doi: 10.1166/jnn.2015.10512
- I Hanno, C Anselmi and K Bouchemal, Polyamide nanocapsules and nano-emulsions containing Parsol MCX and Parsol 1789: In vitro release, ex vivo skin penetration and photo-stability studies, Pharm Res 29(2) 559–573 (2012) doi: 10.1007/s11095-011-0592-5
- S Scalia and M Mezzena, Incorporation in lipid microparticles of the UVA filter, butyl methoxydibenzoylmethane combined with the UVB filter, octocrylene: Effect on photostability, AAPS PharmSciTech 10(2) 384–390 (2009) doi: 10.1208/s12249-009-9217-2
- S Mandal, R Bera, S Das, SK Nayak, A Pramanik and A Patra, Photon harvesting in sunscreen-based functional nanoparticles, Chemphyschem Eur J Chem Phys Phys Chem 16(17) 3618–3624 (2015) doi: 10.1002/cphc.201500780
- N Lapidot, O Gans, F Biagini, L Sosonkin and C Rottman, Advanced sunscreens: UV absorbers encapsulated in sol-gel glass microcapsules, J Sol-Gel Sci Technol 26(1–3) 67–72 (2003) doi: 10.1023/A:1020785217895
- J Yang, C Wiley, D Godwin and LA Felton, Influence of hydroxypropyl-b-cyclodextrin on transdermal penetration and photostability of avobenzone, Eur J Pharm Biopharm 69(2) 605–612 (2008) doi: 10.1016/j.ejpb.2007.12.015
- S Scalia, S Simeoni, A Barbieri and S Sostero, Influence of hydroxypropyl-b-cyclodextrin on photo-induced free radical production by the sunscreen agent, butyl-methoxydibenzoylmethane, J Pharm Pharmacol 54(11) 1553–1558 (2002) doi: 10.1211/002235702207
- MN Chrétien, Supramolecular photochemistry in zeolites: From catalysts to sunscreens, Pure Appl Chem 79(1) 1–20 (2007) doi: 10.1351/pac200779010001
- MN Chrétien, L Migahed and JC Scaiano, Protecting the protectors: Reducing the ciological toxicity of UV sunscreens by zeolite encapsulation, Photochem Photobiol 82(6) 1606–1611 (2006) doi: 10.1562/2006-07-11-RA-967
- MN Chrétien, E Heafey and JC Scaiano, Reducing adverse effects from UV sunscreens by zeolite encapsulation: Comparison of oxybenzone in solution and in zeolites, Photochem Photobiol 86(1) 153–161 (2010) doi: 10.1111/j.1751-1097.2009.00644.x
- E Damiani, P Carloni, C Biondi and L Greci, Increased oxidative modification of albumin when illuminated in vitro in the presence of a common sunscreen ingredient: Protection by nitroxide radicals, Free Radic Biol Med 28(2) 193–201 (2000)
- A Chételat, JH Dresp and E Gocke, Photomutagenesis test development: II. 8-Methoxypsoralen, chlorpromazine and sunscreen compounds in chromosomal aberration assays using CHO cells, Mutat Res 292(3) 251–258 (1993)
- S Schauder and H Ippen, Contact and photocontact sensitivity to sunscreens. Review of a 15-year experience and of the literature, Contact Dermatitis 37(5) 221–232 (1997)
- AC de Groot and JW Weyland, Contact allergy to butyl methoxydibenzoylmethane, Contact Dermatitis 16(5) 278 (1987)
- RJ Motley and AJ Reynolds, Photocontact dermatitis due to isopropyl and butyl methoxy dibenzoylmethanes (Eusolex 8020 and Parsol 1789), Contact Dermatitis 21(2) 109–110 (1989)
- DA Buckley, D O’Sullivan and GM Murphy, Contact and photocontact allergy to dibenzoylmethanes and contact allergy to methylbenzylidene camphor, Contact Dermatitis 29(1) 47 (1993)
- EJH Collaris and J Frank, Photoallergic contact dermatitis caused by ultraviolet filters in different sunscreens, Int J Dermatol 47 suppl 1 35–37 (2008) doi: 10.1111/j.1365-4632.2008.03957.x
- E Parry, D Bilsland and W Morley, Photocontact allergy to 4-tert.butyl-4’-methoxy-dibenzoylmethane (Parsol 1789), Contact Dermatitis 32 251–252 (1995)
- B Berne and AM Ros, 7 Years experience of photopatch testing with sunscreen allergens in Sweden, Contact Dermatitis 38(2) 61–64 (1998)
- European Multicentre Photopatch Test Study (EMCPPTS) Taskforce, A European multicentre photopatch test study, Br J Dermatol 166(5) 1002–1009 (2012) doi: 10.1111/j.1365-2133.2012.10857.x
- S Schauder and H Ippen, Photoallergic and allergic contact dermatitis from dibenzoylmethanes, Photodermatol 3(3) 140–147 (1986)
- T Schmidt, J Ring and D Abeck, Photoallergic contact dermatitis due to combined UVB (4-methylbenzylidene camphor/octyl methoxycinnamate) and UVA (benzophenone-3/butyl methoxydibenzoylmethane) absorber sensitization, Dermatol Basel Switz 196(3) 354–357 (1998)
- JF Nash and PR Tanner, Relevance of UV filter/sunscreen product photostability to human safety, Photodermatol Photoimmunol Photomed 30(2–3) 88–95 (2014) doi: 10.1111/phpp.12113
- LR Gaspar, J Tharmann, PMBG Maia Campos and M Liebsch, Skin phototoxicity of cosmetic formulations containing photo-unstable and photostable UV filters and vitamin A palmitate, Toxicol Vitro Int J Publ Assoc BIBRA 27(1) 418–425 (2013) doi: 10.1016/j.tiv.2012.08.006
- I Karlsson, L Hillerström, A-L Stenfeldt, J Mårtensson and A Börje, Photodegradation of dibenzoylmethanes: Potential cause of photocontact allergy to sunscreens, Chem Res Toxicol 22(11) 1881–1892 (2009) doi: 10.1021/tx900284e
- JA Harrison, SL Walker, SR Plastow, MD Batt, JL Hawk and AR Young, Sunscreens with low sun protection factor inhibit ultraviolet B and A photoaging in the skin of the hairless albino mouse, Photodermatol Photoimmunol Photomed 8(1) 12–20 (1991)
- I Karlsson, K Vanden Broecke, J Mårtensson, A Goossens and A Börje, Clinical and experimental studies of octocrylene’s allergenic potency, Contact Dermatitis 64(6) 343–352 (2011) doi: 10.1111/j.1600-0536.2011.01899.x
- M Avenel-Audran et al, Octocrylene, an emerging photoallergen, Arch Dermatol 146(7) 753–757 (2010) doi: 10.1001/archdermatol.2010.132
- K Atarashi, M Takano, S Kato, H Kuma, M Nakanishi and Y Tokura, Addition of UVA-absorber butyl methoxy dibenzoylmethane to topical ketoprofen formulation reduces ketoprofen-photoallergic reaction, J Photochem Photobiol B 113 56–62 (2012) doi: 10.1016/j.jphotobiol.2012.05.002
- S Forestier, Rationale for sunscreen development, J Am Acad Dermatol 58(5 suppl 2) S133-138 (2008) doi: 10.1016/j.jaad.2007.05.047
- SC Rastogi, UV Filters in sunscreen products—A survey, Contact Dermatitis 46(6) 348–351 (2002)
- DG Beasley and TA Meyer, Characterization of the UVA protection provided by avobenzone, zinc oxide and titanium dioxide in broad-spectrum sunscreen products, Am J Clin Dermatol 11(6) 413–421 (2010) doi: 10.2165/11537050-000000000-00000
- M Morsella, N d’Alessandro, AE Lanterna and JC Scaiano, Improving the sunscreen properties of TiO2 through an understanding of its catalytic properties, ACS Omega 1(3) 464–469 (2016) doi: 10.1021/acsomega.6b00177