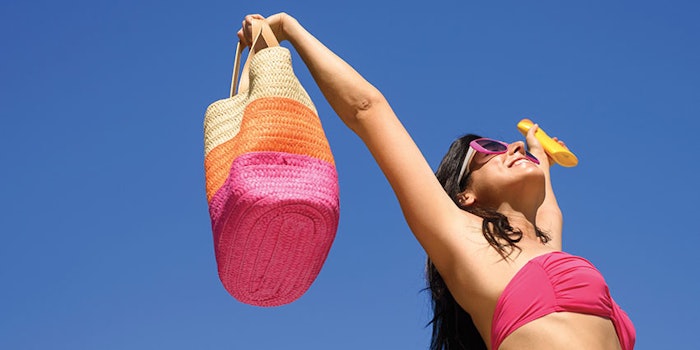
Consumers select sunscreen products based on the labeled sun protection factor (SPF), assuming larger numbers are better. In reality, higher SPF ratings do not necessarily offer significantly higher levels of UV protection. They can even give users a false sense of security, leading them to spend too much time in the sun, which can result in irreversible skin damage. Furthermore, inaccurate SPF values for sunscreens continue to climb—some brands even offer protection higher than SPF 100. Unfortunately, these sunscreens do not provide consumers with the infallible protection they assume they are getting, which puts users at greater risk for sunburn. The need to prevent skin from UV damage is well-understood but the most effective way to achieve this is not. Here, a novel photostabilizer is presented in a test pump sunscreen spray formula as part of a total photoprotection strategy.
Log in to view the full article
Consumers select sunscreen products based on the labeled sun protection factor (SPF), assuming larger numbers are better. In reality, higher SPF ratings do not necessarily offer significantly higher levels of UV protection. They can even give users a false sense of security, leading them to spend too much time in the sun, which can result in irreversible skin damage. Furthermore, inaccurate SPF values for sunscreens continue to climb—some brands even offer protection higher than SPF 100. Unfortunately, these sunscreens do not provide consumers with the infallible protection they assume they are getting, which puts users at greater risk for sunburn. The need to prevent skin from UV damage is well-understood but the most effective way to achieve this is not. Here, a novel photostabilizer is presented in a test pump sunscreen spray formula as part of a total photoprotection strategy.
Clearly, across the globe, not all sunscreens are made equally. The U.S. Food and Drug Administration’s (FDA’s) new broad spectrum rule is still insufficient compared with Europe, where UVA protection is required to rise in proportion with SPF. Furthermore, revised EU guidelines mandate a minimum level of UVA protection in terms of SPF. In this case, the UVA protection factor is measured either in vivo by persistent pigment darkening (PPD), or in vitro by Cosmetic Europe’s methods and guidelines—which must yield results that are at least one-third of the SPF in vivo value.1
As late as 2011, the FDA enacted its first-ever sunscreen rules, which require sunscreens claiming “broad spectrum” protection to pass a critical wavelength (370 nm) test. As a result, nearly all broad-spectrum sunscreens sold today include either zinc oxide or avobenzone, which successfully filter UV rays. This is a positive change in the U.S. market.
It is worth mentioning that a comprehensive literature review of all sunscreen studies between 1966 and 2003 found no evidence of sunscreens increasing melanoma risk.2, 3 In fact, recent research shows more convincingly than ever that sunscreens protect against melanoma. In 2011, a groundbreaking study of 1,621 Australians found that regular sunscreen users reduced their incidence of melanoma by 50–73%.4 This landmark trial in humans was the first to show a significant and definitive benefit from the continuous use of sunscreens.
Consumer awareness of the need for sunscreens has grown, which is evidenced by the dramatic increase in use of inorganic sunscreen filters—especially zinc oxide and titanium oxide—in the U.S. market. In addition, zinc oxide and titanium dioxide tend to rate well with consumers since they are perceived to be natural; however, this is debatable.
While such inorganic sunscreens are photochemically stable in sunlight, it is important to note they can become photoreactive if they are not properly coated. Silica-based coatings are the most effective and can be formulated in acidic pH.5 Generally speaking, however, zinc and titanium dioxide offer a good balance of protection against both UVA and UVB rays. Regarding nanoparticle sizes of these materials, the overall weight of scientific evidence suggests these insoluble particles pose little or no risk to human health; indeed, any risk is far outweighed by their benefits.6
Considering application rate, the FDA recommends applying 2 mg/cm2 of sunscreen to achieve maximum benefits. Reapplication is necessary every two hours as well as after sweating, toweling off, bathing and swimming.7 The product label should highlight these instructions since insufficient application and failure to reapply are the main reasons sunscreens fail; misuse creates a mismatch between the labeled SPF and actual SPF delivered upon application to skin and exposure to sunlight.8, 9
Taking these challenges and considerations together, this article describes a strategy to formulate a safe and effective pump sunscreen spray. It utilizes a novel photostabilizer, i.e., 3-(3,4,5-trimethoxybenzylidene)-2,4-pentanedione (INCI: Trimethoxybenzylidene Pentanedione; TMBP), which was developed through careful consideration of the atom economy—i.e., the strategy that every atom plays a role in achieving the objective. The efficacy of TMBP was compared with other photostabilizers in the same base formula. Note that in order to achieve broad applications of TMBP for personal care as well as other industries, no free phenolic OH groups were included as they impart color to the product.
Broad-spectrum Protection and Photostability
A key focus for designing sunscreen products is to select a combination of UV filters that delivers broad-spectrum protection with maximum efficiency. Claiming broad-spectrum protection in the United States requires both an SPF ≥ 15 and critical wavelength ≥ 370 nm. As noted, Europe sets a much higher bar, requiring UVA protection to rise in proportion with SPF. The European Cosmetics Association, Cosmetics Europe, describes an in vitro method for this measurement.10
Combining UV filters can lead to synergies for desired performance and photostability; however, accelerated decomposition also can occur if they photo-react with one another.
The presence of two UV filters can lead to synergistic effects in terms of both the final performance and photostabilization of the active principles. However, this also may result in an accelerated decomposition if a photoreaction occurs between the two components.11 Consequently, photostability is imperative if the product is to achieve maximum efficiency, as it has a significant impact on the SPF and, particularly, protection in the UVA region (320–400 nm).
While a broad range of globally approved organic UVB filters is available, 4-tert-butyl-4'-methoxydibenzoylmethane (avobenzone, see Figure 1) is the only worldwide approved organic UVA filter. For the purposes of the present work, several sunscreen ingredients were ruled out due to questions over their safety (see Sunscreen Ingredients of Documented Concern).
Unfortunately, it is well-documented that under certain conditions, exposure to UV radiation will cause avobenzone to photodegrade. Specifically, avobenzone shows a strong absorption maximum at about 355–360 nm, which decreases under UV irradiation, resulting in a loss of the UVA protection.12, 13 Avobenzone photo-instability has been shown to be sensitive to the properties of the solvent environment; it is stable in polar protic solvents whereas it is highly photo-unstable in aprotic polar and nonpolar solvents.14–16
Mturi and Martincigh showed that avobenzone is photostable in the polar protic solvent methanol but photo-isomerized in the polar aprotic solvent dimethysulfoxide and photodegraded in the nonpolar solvent cyclohexane.16 Photo-isomerization occurred only in the presence of oxygen, whereas photodegradation occurred irrespective of oxygen. This is important to understand in order to achieve the desired photostability in sunscreen formulations. Unfortunately, many commercial sunscreens still lack the desired photostability.17, 18
It is also worth noting there are clinical reports of photoallergy19, 20 and phototoxicity attributed to avobenzone.21 Its photoallergic potential may be due to the photodegradation products generated following UV exposure;22 for example, Karlsson et al. showed the formation of arylglyoxals and benzils, and arylglyoxals were characterized as strong sensitizers in a mouse local lymph node assay.22
Considering this photodegradation and photo-allergic potential, it is clear that photostabilizing avobenzone would greatly reduce the likelihood of a photo-allergic event; although in a recent study, Nash and Tanner elegantly reviewed the relevance of sunscreen product photostability to human safety.23 They found that photo-unstable UV filters or sunscreen products may not manifest as health risks under conditions of use. Still, improvements in sunscreen photostability continue as a key objective to increase sunscreen efficacy.
Selecting an Avobenzone Photostabilizer
Triplet-triplet quenchers have been used in sunscreens for more than a decade now and have opened up a new avenue for photostabilization of avobenzone.24 To ensure maximum efficiency, a range of triplet state quenchers often is used, including the UVB filter octocrylene (OCR, see Figure 2a), diethylhexyl-2,6-napthalate,25 ethylhexyl methoxycrylene (EMC, see Figure 2b),26 diethylhexylsyringylidenemalonate (DESM, see Figure 2c)27 and the newly developed TMBP (see Figure 2d).28 These materials were used in the present study to compare their effects.
Selecting UVB Sunscreens
As stated, concerns have been expressed over the safety of some filters including octocrylene (OCR), benzophenone-3 (BP3) and octyl methoxycinnamate (OMC). These were therefore ruled out for the test spray, leaving just two UVB options for the U.S. market: homosalate (HMS, see Figure 3a) and octisalate (OS, see Figure 3b). Since these two UVB sunscreens are not as effective as desired, the selection of aforementioned photostabilizers, SPF boosters and other ingredients that improve avobenzone’s photostability are key to consider. In fact, these supporting ingredients essentially are the only viable options available to formulators targeting the U.S. market.
In vivo SPF Boosters
Recent years have seen the launch of several ingredients promoted as SPF boosters. These ingredients can significantly increase the in vivo SPF; however, alone, they do not contribute meaningfully to SPF. Boosting SPF is possible either by optimizing the efficacy of the UV-absorbing system, or by improving the film-forming properties of the product during spreading. This helps formulators to develop higher SPF products while also using lower UV filter levels.
The concept of SPF boosting was first introduced with solvents and solubilizers that dissolved solid UV filters such as avobenzone more efficiently; examples of good solubilizers for avobenzone are given in Table 1. These not only aid the dissolution of avobenzone, but they also affect the absorbing patterns, resulting in better coverage, which translates to an improved in vivo SPF.29
Several film-forming polymers also claim SPF-boosting and improved photostability properties;30–32 some oil-soluble examples are given in Table 2. For spray sunscreens, these polymers typically are used in a range of 1–3% (actives basis), and most increase UV absorption by maintaining a uniform sunscreen film thickness on skin over time.
Materials and Methods
As noted, the chosen materials were put to the test in pump sunscreen sprays. Materials were used as received by suppliers and included: avobenzone, HMS, OS and DESMa; OCRa and EMCb also were used for comparison purposes. All other ingredients are shown in Formula 1. Here, HMS, OS, avobenzone and TMBPc were incorporated, and avobenzone and TMBP levels were adjusted to obtain an SPF of 50+. Photostability studies were carried out with TMBP, or OCR, EMC or DESM in its place.
Avobenzone stability is sensitive to the properties of the solvent environment.
In vitro Photostability
Transmittance was measured with a UV transmittance analyzerd. All irradiations were performed using a solar stimulator equipped with a 150 W xenon arc lamp. The output of the solar simulator was checked with a solar irradiance metere. Two slides were prepared for each sunscreen formulation tested. A dose of 100 µL cm-2 of the samples (product dose of 2 µL cm-2) was applied by hand (not sprayed) evenly on 6.2 × 8.0 cm sections of a test substratef. Immediately after application, the product was spread evenly with similar amount of force using a gloved finger. The film was then left to dry for 15 min.
Five consecutive UV transmission measurements on five separate areas were taken on the slide immediately after drying. The initial reading before irradiation was calculated by taking five separate readings from each slide. These 10 readings—i.e., five from each slide—were then averaged to obtain the base-line static SPF value.
The center points of each slide were irradiated with 10 MEDs, readings were taken, averaged and reported. Likewise, the center points on the same slides were exposed to a total of 20 MEDs and 40 MEDs, and data was obtained and reported accordingly. The reference and sample pieces used in the study were from the same sheet of hydrated test substrate.
In vivo SPF
The static SPF on human skin was determined based on the procedure described by the U.S. Food and Drug Administration 21 CFR Parts 201 and 310.33, 34 A xenon arc solar simulator was used as the UV source. Note that five subjects were tested rather than the minimum of 10 cited in these references.
Free Radical Inhibition and Human Safety
The extent to which free radicals cause damage in underlying skin mechanisms has recently become more elucidated. Thus, the activity of TMBP on free radicals was assessed. However, this material is not a conventional antioxidant, working by scavenging radicals; rather, it works by reducing the formation of free radicals. Therefore, its effects were measured by its inhibitory activity against nicotinamide adenine dinucleotide phosphate oxidase (NADPH Oxidase or NOX). This experiment was carried out mainly following the protocols of Royall and Ischiropoulos, with some minor changes,35 and Valencia and Kochevar.36
Adult human dermal fibroblasts were grown in a 96-well plate supplemented with DMEM 5% calf serum to subconfluency and the medium was replaced with PBS. Cells were exposed to experimental conditions for 20 min, after which 50 µM 2’,7’-dichlorofluorescin diacetate (DCFH-DAg) was added and the plate—except for the non-UVA exposed control—was irradiated at 5 mW/cm2 for 12 min using a UVA lamph for a total irradiation dose of 3.6 J/cm2.36
DCFH-DA is a nonpolar compound that readily diffuses into cells where it is hydrolyzed (oxidized) to the nonfluorescent polar derivative DCFH and thereby trapped within the cells. In the presence of H2O2, DCFH is oxidized to the highly fluorescent DCF. This hydrolysis is proportional to the concentration of intracellular peroxides and can be detected in living cells by fluorometry at ex/em 488/530 nm.35 Reactive oxygen species (ROS) were then quantified using a fluorimeterj at three different points in time. Each experimental test was repeated a minimum of seven times. Regarding human safety, human repeat insult patch testing (HRIPT) of TMBP also was performed, described elsewhere.37
Eco-toxicity Profile
As environmental safety is an important consideration, TMBP also was evaluated for biodegradability and aquatic toxicity. Biodegradability was determined using the OECD 3011D protocol at a concentration of 2 mg/L in mineral medium inoculated with secondary effluent of a treatment plant (1 ml/L). Potassium hydrogen phthalate was used as control. Mineral medium inoculated (1ml/L) without TMBP or potassium hydrogen phthalate served as the inoculum blank. Short-term toxicity to aquatic invertebrates (Daphnia sp.) also was assessed, based on OECD 202.
Discussion
As stated, the key focus in developing sunscreen products is to select a combination of UV filters that delivers the intended broad-spectrum protection with maximum efficiency. Consequently, photostability is imperative in order for the product to achieve and maintain maximum efficiency, as it has a significant effect on the SPF—particularly for the UVA region (320–400 nm).
In this work, the photostabilizer and in vivo SPF booster TMBP28 was evaluated in sunscreen sprays containing two UVB absorbers, HMS and OS, and the UVA absorber avobenzone. A key advantage to using ethanol in the spray sunscreen is avobenzone’s aforementioned stability in it;1 furthermore, this system requires no preservation.
The efficacy of a sunscreen is defined by two parameters: the SPF and UVA protection parameter. The SPF is provided by in vivo testing in a panel of human subjects, although different protocols for determining SPF are used in different countries/regions. And indeed, differences in the details of the SPF test method can make significant differences in the results. For example, the U.S. protocol tends to overestimate the SPF number versus the protocol used in Europe. The present authors used the FDA protocol for determining the in vivo SPF of the test products.
UVA-irradiated fibroblasts revealed ROS continued to increase 1 min to 6 min post-irradiation, suggesting a cascade or enzyme-mediated effect.
Results: SPF and Critical Wavelength
The in vivo SPF and critical wavelength of variations of Formula 1 are summarized in Table 3. Levels of avobenzone at 3% and TMBP at 2.5%, along with HMS at 10% and OS at 5%, were required to obtain an in vivo SPF > 50. This formulation was very effective as it provided an SPF of 56 with only 18% sunscreen, meaning an SPF yield of 3.1 to percentage of sunscreen. Also, the critical wavelengths of all four formulations were > 375 nm and hence qualified as broad-spectrum sunscreens.
Compared with the SPF of the formula without stabilizer, TMBP boosted the in vivo SPF of the formulation by 75% (SPF 32 to 56), whereas the other stabilizers provided boosts from ~25% to ~ 34%. This is because TMBP also absorbs in the UVB region, and since SPF is a measure of efficacy in this region, TMBP can boost the SPF. Interestingly, the ability to boost SPF in vivo was observed only when TMBP was used with other UV absorbers while alone it makes no contribution to SPF.28
In the sunscreen spray with no photostabilizer, it was apparent that increasing exposure to solar simulated irradiation caused a marked loss of photoprotection in the UVA region. Here, 20 MED showed a 50% loss and 40 MED, a 73% loss (see Figure 4a). Avobenzone is well-known to photodegrade, so this is not a surprising result. And although less severe, photodegradation also was apparent with DESM; 40 MED registered a loss of 45%, compared with the control (see Figure 4b).
In the presence of TMBP, a marked improvement in photostability occurred in the UVA region. Here, a minimal loss of just 4% at 40 MED was observed, and no loss at 20 MED (see Figure 4c). TMBP was thus able to prevent the photodegradation of avobenzone.17 TMBP was equally effective as OCR and EMC (see Figure 4d and Figure 4e, respectively).
Results: Antioxidant Activity and Human Safety
In response to free radicals, TMBP provided a key advantage in that it inhibited their excessive formation, as induced by the over-production of NOX. The NOX family includes seven unique members that are now considered the primary regulated sources of ROS.38 These enzymes are expressed in diverse cells and tissues, and their products are essential to several physiological settings. For example, they share the capacity to transport electrons across the plasma membrane and to generate superoxide and other downstream ROS.
Knockout mouse models have been instrumental in identifying the physiological functions of NOX enzymes and the impact of their pharmacological targeting on disease progression.39 NOX represents the first step in the oxidative stress cascade. Keratinocytes and fibroblasts both generate ROS in response to UV light,40 which involves NOX enzymes. NOX-derived ROS are also involved in the regulation, expression and/or activation of matrix metalloproteases MMPs,38 which are well-known to cause damage to collagen, elastin and other skin proteins.
Recently, Raad et al.41 explored the role of NOX1 in photocarcinogenesis and its importance in the DNA damage repair network. Their work suggested a transient surge of ROS may serve as a priming signal to trigger a series of redox reactions that further stimulate cellular defense mechanisms, including the DNA damage response.
Results of the preliminary work described here using fibroblasts (UVA irradiation 3.6 J/ m2) revealed that ROS continued to increase from 1 min to 6 min post-irradiation, suggesting a cascade/or enzyme-mediated effect (see Figure 5). ROS started to subside 11 min post-irradiation due to the lack of continuing stimulation. However, a nearly eightfold decrease in radical formation was observed using only 20 µg/mL of TMBP. Thus, TMBP appears to inhibit excessive radical formation induced by the over-production of NOX due to UV exposure.
In terms of human safety, as noted, HIRPT was performed in 103 subjects using 2% and 5% dilutions of TMBP in corn oil and isosorbide dicaprylate, respectively. Results showed TMBP to be a non-primary skin irritant and non-primary skin sensitizer, and no adverse effects or reactions were observed. The material also was found to be non-phototoxic and non-mutagenic.37
In comparison, OCR, while an effective avobenzone stabilizer, is emerging as a potential photo-allergen as it has been connected with increasing reports of positive patch and photopatch test reactions.42 EMC is a methoxy analog of OCR, and while not explicitly identified as a sensitizer, could also pose similar safety concerns.
Results: Ecological Safety
Many UV filters represent some risk to the environment due to their lipophilicity and hence, have poor biodegradability. They can end up in sewage sludge during waste water treatment and accumulate in river sediments and biota at concentrations ranging from nano to µg/g. The eco-toxicological effects of widely used organic UV filters can be found at science.gov.43
However, since it is the only UVA filter approved by the FDA, avobenzone is the only choice for formulators targeting the U.S. market. While avobenzone is not readily biodegradable, it is photodegradable and it is neither bio-accumulative nor toxic to fish or algae.44 Likewise, the UV filter HMS is not readily biodegradable, bio-accumulative or toxic to fish or algae. The UV filter OS, however, is readily biodegradable and not toxic to fish or algae.45 Interestingly, avobenzone and OS have not been found to bleach coral, whereas BP-3 and OMC have.46
For the present study, as noted, the biodegradability of TMBP was determined following the OECD protocol 3011D. The biodegradability of TMBP was found to be -2.04%, 2.30%, 8.18% and 30.0% (based on ThOD, i.e., 1.957 mg O2/g) on days 7, 14, 21 and 28, respectively. Extrapolation of this data revealed TMBP is estimated to degrade fully in approx. six weeks (see Figure 6).
In relation to safety for aquatic vertebrates, after 48 hr, TMBP registered an EC50 > 25 mg/L, deeming it non-toxic to Daphnia sp. Toxicity to aquatic algae was assessed by OECD 201. After 72 hr, it registered an EC50 >25 mg/L, indicating TMBP is non-toxic to algae (Pseudokirchnerella subcapitata).
Conclusion
The present article in no way attempts to provide a comprehensive review of all the safety and eco-toxicity data available for sunscreens in the literature. However, authors have analyzed key data available to pinpoint the challenges formulators face to develop safe and effective sunscreen products for the U.S. market.
Based on the presented in vitro photostability and in vivo SPF data, one can conclude that TMBP can provide safe, eco-friendly and photostable broad spectrum protection (SPF 50+), as demonstrated in a sunscreen spray and earlier, in a lotion.28 It is worth noting the ethanol content in the test spray should be maintained in the range 40–45% in order to achieve the in vivo SPF of 50+. In relation, since ethanol sprays tend to make skin feel dry, the addition of ingredients such as isosorbide dicaprylate can provide a soft and smooth skin feel.36
TMBP also was found to reduce the upstream oxidative stress cascade, presumably via the NOX family. Finally, a significant synergistic boost (75%) to the in vivo SPF was observed using TMBP (2.5%) with the globally approved UV absorbers avobenzone (3%), HMS (10%) and OS (5%). Taken together, these facets offer a complete sunscreen protection strategy array.
References
- Colipa method for in vitro determination of UVA protection, European Cosmetics Association (2009)
- M Huncharek and B Kupelnick, Use of topical sunscreens and the risk of malignant melanoma: A meta-analysis of 9,067 patients from 11 case-control studies, Am J Public Health 92 1173-1177 (2002)
- LEB Freeman, MJ VanBeek et al, Sunscreen use and the risk for melanoma: A quantitative review, Ann Intern Med 139 966-978 (2003)
- AC Green, GM Williams, V Logan and GM Strutton, Reduced melanoma after regular sunscreen use: Randomized trial follow-up, J Clin Oncol 29(3) 257-263 (2011)
- TG Smijs and S Pavel, Titanium dioxide and zinc oxide nanoparticles in sunscreens: Focus on their safety and effectiveness, Nanotechnol Sci Appl 4 95–112 (2011)
- GJ Nohynek and EK Dufour, Nano-sized cosmetic formulations or solid nanoparticles in sunscreens: A risk to human health? Arch Toxicol 86(7) 1063-1075 (2012)
- DB Buller et al, Compliance with sunscreen advice in a survey of adults engaged in outdoor winter recreation at high-elevation ski areas, J Am Acad Dermatol 66 63–70 (2012)
- MS Latha et al, Sunscreening Agents, J Clin Aesthet Dermatol 6(1) 16–26 (2013)
- R Novick, G Anderson, E Miller, D Allgeier and K Unice, Factors that influence sunscreen application thickness and potential preservative exposure, Photodermatol Photoimmunol Photomed 31(4) 212-223 (2015)
- Ibid Ref 1
- V Lhiaubet-Vallet, M Marin, O Jimenez, O Gorchs, C Trullas, and MA Miranda, Filter-filter interactions. Photostabilization, triplet quenching and reactivity with singlet oxygen, Photochemistry and Photobiological Sciences 9(4) 552-8 (2010)
- H Gonzenbach, TJ Hill, and TG Truscott, The triplet energy levels of UVA and UVB sunscreens, J Photochem Photobiol, B 16(3-4) 377-379 (1992)
- W Schwack, and T Rudolph, Photochemistry of dibenzoyl methane UVA filters Part 1. J Photochem and Photobiol B: Biology 28 229-234 (1995)
- NM Roscher, MKO Lindemann, SB Kong, CG Cho and P Jiang, Photodecomposition of several compounds commonly used as sunscreen agents, J Photochem and Photobiol A: Chemistry 80(1-3) 417-421 (1994)
- I Andrae et al, A UVA filter (4-tert-butyl-4’-methoxydibenzoylmethane): Photoprotection reflects photophysical properties, J Photochem and Photobio B: Biology 37 147-150 (1997)
- GJ Mturi and BS Martincigh, Photostability of the sunscreening agent 4-tert-butyl-4’-methoxydibenzoylmethane (avobenzone) in solvents of different polarity and proticity, J Photochem Photobiol A: Chemistry 200 410-420 (2008)
- H Gonzalez et al, Photostability of commercial sunscreens upon sun exposure and irradiation by ultraviolet lamps, BMC Dermatology 7 1-9 (2007)
- H Maier, G Schauberger, BS Martincigh, K Brunnhofer and H Hönigsmann, Ultraviolet protective performance of photoprotective lipsticks: Change of spectral transmittance because of ultraviolet exposure, Photodermatol, Photoimmunol and Photomed 21(2) 84-92 (2005)
- S Schauder and H Ippen, Photoallergic and allergic contact dermatitis from dibenzoylmethanes, Photodermatol 3(3) 140-7 (1986)
- RJ Motley and AJ Reynolds, Photocontact dermatitis due to isopropyl and butyl methoxy dibenzoylmethanes (Eusolex 8020 and Parsol 1789), Contact Derm 21(2) 109-10 (1989)
- LR Gaspar, J Tharmann, PM Maia Campos and M Liebsch, Skin phototoxicity of cosmetic formulations containing photo-unstable and photostable UV-filters and vitamin A palmitate, Toxicology In vitro 27(1) 418-25 (2013)
- I Karlsson, L Hillerstrom, AL Stenfeldt, J Martensson and A Borje, Photodegradation of dibenzoylmethanes: Potential cause of photocontact allergy of sunscreens, Chem Res in Toxicol 22 1881-92 (2009)
- JF Nash and PR Tanner, Relevance of UV filter/sunscreen product photostability to human safety, Photoderm, Photoimmunol and Photomed 30(2-3) 88-95 (2014)
- CA Bonda, The photostability of organic sunscreen actives, in Sunscreens, CRC Press, Boca Raton, FL USA (2005) pp 321-49
- C Bonda and D Steinberg, A new photostabilizer for full spectrum sunscreens, Cosm & Toil 115(6) 37-45 (2000)
- C Bonda and A Pavlovic, Singlet quenching proves faster is better for photostability, Cosm & Toil 125(2) 40-8 (2010)
- RK Chaudhuri, Z Lascu, G Puccetti, AA Deshpande and SK Paknikar, Design of a photostabilizer having built-in antioxidant functionality and its utility in obtaining broad-spectrum sunscreen formulations, Photochem and Photobio 82(3) 823-8 (2006)
- RK Chaudhuri, MA Ollengo, P Singh and BS Martincigh, 3-(3,4,5-Trimethoxybenzylidene)-2,4-pentanedione: Design of a novel photostabilizer with in vivo SPF boosting properties and its use in developing broad-spectrum sunscreen formulations, Int J Cosmet Sci 39 25-35 (2017)
- N Shaath, SPF boosters and photostability of ultraviolet filters, available at happi.com/contents/view_features/2007-10-01/spf-boosters--photostability-of-ultraviolet-f (accessed Mar 16, 2018)
- D Prettypaul and H Fares, Microscopic evaluation of polymeric film properties of anhydrous sunscreen compositions and their relation to absorption and water resistance, J Cosmet Sci 63(3) 213-221 (2012)
- JA Davis, D Petersen and D Li, Use of film-forming polymers for increased efficacy in sunscreens, J Cosmet Sci 58(5) 568–569 (2007); A Hunter and M Trevino, Film-formers enhance water resistance and SPF in sun care products, Cosm & Toil 51–55 (2004)
- JA Royall, H Ischiropoulos, Evaluation of 2’,7’-dichlorofluorescin and dihydrorhodamine 123 as fluorescent probes for intracellular H2O2 in cultured endothelial cells, J Physiol Pharmacol 2011 62(2) 229 (2011)
- www.accessdata.fda.gov/scripts/cdrh/cfdocs/cfCFR/CFRSearch.cfm?CFRPart=201 (Accessed on Mar 16, 2018)
- accessdata.fda.gov/scripts/cdrh/cfdocs/cfcfr/CFRSearch.cfm?CFRPart=310&showFR=1&subpartNode=21:5.0.1.1.2.4 (Accessed on Mar 16, 2018)
- A Valencia and IE Kochevar, Nox1-based NADPH oxidase Is the major source of UVA-induced reactive oxygen species in human keratinocytes, J Invest Dermatol 128 214 (2008)
- RK Chaudhuri and K Bojanowski, Improvement of hydration and epidermal barrier function in human skin by a novel compound isosorbide dicaprylate, Int J Cosmet Sci 39(5) 518-526 (2017)
- https://echa.europa.eu/fr/registration-dossier/-/registered-dossier/10239 (Accessed on Mar 16, 2018)
- K Bedard and KH Krause, The NOX family of ROS-generating NADPH oxidases: Physiology and pathophysiology, Physiol Rev 87(1) 245-313 (2007)
- G Sirokmány, Á Donkó and M Geiszt, Nox/Duox family of NADPH oxidases: Lessons from knockout mouse models, Trend in Pharmacol Sci 37(4) 318–327 (2016)]
- SM Beak, YS Lee and JA Kim, NADPH oxidase and cyclooxygenase mediate the ultraviolet B-induced generation of reactive oxygen species and activation of nuclear factor-kappaB in HaCaT human keratinocytes, Biochimie 86 425–429 (2004)
- H Raad et al, NADPH oxidase-1 plays a key role in keratinocyte respsonses to UV radiation and UVB-induced skin carcinogenesis, J Invest Dermatol 137 1311-1321 (2017)
- M Avenel-Audran, H Dutartre, A Goossens, Octocrylene, and emerging photoallergen, Arch Dermatol 146(7) 753-757 (2010)
- science.gov/topicpages/o/organic+uv+filters.html
- M Schlabach et al, Environmental screening of selected organic compounds 2008, Norwegian Pollution Control Authority, SPFO-rapport 1046/2009
- https://echa.europa.eu//registrationdossier/-/registered-dossier
- ncbi.nlm.nih.gov/pmc/articles/PMC2291018/